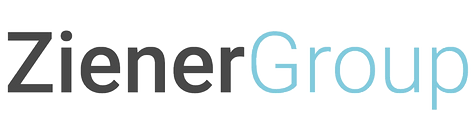
Self-assembled functional materials

Current Research Topics
Our research is directed towards structure and morphology controlled self-assembling systems. Organic synthesis allows tailoring of the molecular building blocks and programming their capability for self-assembly by fine-tuning the intermolecular interactions. Those interactions are based on rather weak forces like van-der-Waals, π-π, and (weak) C-H…N interactions. Self-assembly takes place in molecular mono- and multilayers, bulk phases, and in solution. The generated assemblies represent functional units on the nanometer scale. Besides their ability for self-assembly oligopyridine molecules prove to be versatile building blocks for TADF materials for improved OLEDs.
In another self-organising approach (hetero)adamantane containing polymers are used as templates for the synthesis of nanodiamonds. Besides molecular self-assembly we are interested in colloidal systems like (nano)emulsions stabilized by various solid entities (Pickering-type stabilization) and structure formation therein.
More details on these topics can be found below (Research CV). For an overview on other topics in our Institute press the Read More button.
The team page is here.

Research CV
Research topics change, evolve over time and depend heavily on various intrinsic and extrinsic parameters, such as individual interests shaped by rational and emotional influences, encounters with influential researchers, general funding policies, etc. To better understand how the main themes in my group have developed, I will give a brief historical overview of my research life.
​
Already at the beginning of my studies at the University of Tübingen (1986-1995), I was fascinated by the creative toolbox of organic chemistry and decided to focus on organic synthesis. Although I was very intrigued by natural product synthesis, it turned out that the combination of preparative chemistry with physico-chemical aspects broadened the perspective considerably, which seemed much more attractive to me than specialising exclusively in a single branch of classical chemistry. For this reason, I have spent my entire research life on the synthesis of organic materials. During my diploma thesis and PhD at the University of Tübingen (1992-1995), I worked in Michael Hanack's group on the synthesis of phthalocyaninato and related metal complexes as potential organic semiconductors for optoelectronic applications.[1]
Then I moved to the Max Planck Institute for Polymer Research in Mainz, Germany, as a postdoc and joined Gerhard Wegner's group with Adelheid Godt's subgroup in 1996. Here I worked on the synthesis of oligo(phenyleneethynylene)s as rod coil systems.[2] In a second postdoctoral stay with Jean-Marie Lehn at the Université Louis Pasteur in Strasbourg, France (1997-1998), I deepened my knowledge of supramolecular chemistry and synthesised grid-type metal complexes by self-assembly.[3] The highly motivating supervisor and the environment provided by the international working group and the wonderful European city laid the foundation for my involvement with oligopyridine chemistry in various aspects, which continues to this day (see below and current topics). After a stay at the Institute for New Materials in Saarbrücken (1998-2000), where I was able to learn a lot about the way of thinking in industry through industrial projects, I decided to stay in science and joined the research group of Martin Möller at the University of Ulm, Germany in 2000.
In Ulm, I first started my independent research on oligothiophenes as semiconductors for organic field-effect transistors (OFETs). In this field, we were able to present the longest oligothiophene ever synthesised without substituents in the 3,4-position,[4] a concept for predicting the thermal and electrical properties of oligothiophenes with end caps by a simple bulkiness parameter[5] and a monolayer OFET made of a septithiophene that can be processed from solution to form liquid crystalline phases.[6] The oligothiophenes are ideally suited for the study of (intracellular) self-organisation and supramolecular polymerisation.[7]
In parallel, I continued research with oligopyridines (so-called bis-terpyridines BTPs) and related compounds, focusing on their two-dimensional self-assembly. This was done in collaborative projects within the framework of the Collaborative Research Centre 569 (Hierarchical Structure Formation). Due to subtle intra- and intermolecular C-H...N hydrogen bonds, the BTPs show a rich self-assembly behaviour not only at the gas/solid interface,[9] but also at the liquid/solid interface,[8] which is solvent and concentration dependent.[8c] The resulting structures can be employed as hosts for the uptake of metal atoms[10] and large organic guest molecules[11] and can even be manipulated with the tip of the scanning tunneling microscope (STM).[11a] Further functionalization of the BTPs[12] makes self-assembly more complex with competing intermolecular interactions (H bonds, pi-pi).[13] This allows the self-assembly of a given BTP to be viewed as supramolecular chain growth (co)polymerization with similar statistics to bulk copolymerization.[13b]
With the change at the head of our Institute in 2003 I entered the field of colloidal chemistry, in particular mini- or nanoemulsions, where the droplets can be considered as independent nanoreactors. They can be used as versatile systems for the study of interfacial chemistry and the preparation of nanoobjects with different morphologies (particles, capsules etc.). The combination of the mesoscale of droplets with the self-assembly of dye molecules[14] opens the way to defined (inorganic) small nanoparticles and -capsules.[15]
Another change of institute director in 2018 led to a close collaboration in the field of thermally activated delayed fluorescence (TADF) materials. TADF has proven to be a robust concept boosting the external quantum efficiency (EQE) of well-established OLED materials beyond the 25% limit based on spin statistics. It exploits the dark triplet states through enhanced spin orbital (SOC) and vibronic coupling (VC), e.g., in twisted donor-acceptor molecules. Here, the highly versatile electron-deficient oligopyridines are combined with donor units.[13b, 16]
In 2018 I also started a new field on the controlled synthesis of nanodiamonds (NDs). NDs are very attractive because they can be doped with heteroatoms such as nitrogen resulting in so-called nitrogen vacancy (NV) centres that act as stable (non-bleaching, non-blinking) light emitters. Normally, NDs are synthesized by detonation, a poorly controlled process. We use adamantane-based polymers as templates to synthesize NDs in a much more controlled way.[17]
The wealth of different research topics could and can only be managed with the enthusiastic commitment of numerous group members, past and present. I am very grateful for the support of all the young people with whom I have been able to work and will be able to work with in the future.
​
Only a small number of publications are cited in the text above. A complete list of publications can be found here.
​
[1] a) U. Ziener, N. Fahmy, M. Hanack, Chem. Ber. 1993, 126, 2559-2563; b) U. Ziener, M. Hanack, Chem. Ber. 1994, 127, 1681-1685; c) U. Ziener, K. Dürr, M. Hanack, Synth. Met. 1995, 71, 2285-2286.
[2] a) U. Ziener, A. Godt, J. Org. Chem. 1997, 62, 6137-6134; b) H. Kukula, U. Ziener, M. Schöps, A. Godt, Macromolecules 1998, 31, 5160-5163.
[3] a) U. Ziener, E. Breuning, J.-M. Lehn, E. Wegelius, K. Rissanen, G. Baum, D. Fenske, G. Vaughan, Chem.-Eur. J. 2000, 6, 4132-4139; b) E. Breuning, U. Ziener, J.-M. Lehn, E. Wegelius, K. Rissanen, Eur. J. Inorg. Chem. 2001, 1515-1521; c) U. Ziener, J.-M. Lehn, A. Mourran, M. Möller, Chem.-Eur. J. 2002, 8, 951-957; d) M. Ruben, U. Ziener, J.-M. Lehn, V. Ksenofontov, P. Gütlich, G. B. M. Vaughan, Chem.-Eur. J. 2005, 11, 94-100.
[7] a) D. Y. W. Ng, R. Vill, Y. Wu, K. Koynov, Y. Tokura, W. Liu, S. Sihler, A. Kreyes, S. Ritz, H. Barth, U. Ziener, T. Weil, Nat. Commun. 2017, 8, 1850; b) U. Ziener, H. Barth, Nachr. Chem. 2018, 66, 1146-1149; c) R. Vill, J. Gülcher, P. Khalatur, P. Wintergerst, A. Stoll, A. Mourran, U. Ziener, Nanoscale 2019, 11, 663-674.
[8] a) C. Meier, U. Ziener, K. Landfester, P. Weihrich, J. Phys. Chem. B 2005, 109, 21015-21027; b) C. Meier, K. Landfester, U. Ziener, J. Phys. Chem. C 2009, 113, 1507-1514; c) C. Meier, M. Roos, D. Künzel, A. Breitruck, H. Hoster, K. Landfester, A. Gross, R. J. Behm, U. Ziener, J. Phys. Chem. C 2010, 114, 1268-1277; d) D. Caterbow, D. Künzel, M. G. Mavros, A. Groß, K. Landfester, U. Ziener, Beilstein J. Nanotechnol. 2011, 2, 405-415.
[10] A. Breitruck, H. E. Hoster, C. Meier, U. Ziener, R. J. Behm, Surf. Sci. 2007, 601, 4200-4205.
[11] a) C. Meier, K. Landfester, D. Künzel, T. Markert, A. Groß, U. Ziener, Angew. Chem. Int. Ed. 2008, 47, 3821-3825; b) C. Meier, K. Landfester, U. Ziener, J. Phys. Chem. C 2008, 112, 15236-15240.
[12] a) Y. Dai, B. Eggers, M. Metzler, D. Künzel, A. Groß, T. Jacob, U. Ziener, Phys. Chem. Chem. Phys. 2016, 18, 6668-6675; b) B. Eggers, U. Ziener, Chem. – Eur. J. 2018, 24, 14968-14973.
[13] a) F. D. Goll, A. L. Schleper, A. J. C. Kuehne, U. Ziener, J. Phys. Chem. C 2020, 124, 20213-20221; b) F. D. Goll, G. Taubmann, U. Ziener, Angew. Chem. Int. Ed. Engl. 2022, e202117580.
[14] S. Sihler, U. Ziener, Langmuir 2017, 33, 1239-1247.
[15] a) S. Sihler, P. L. Nguyen, M. Linden, U. Ziener, ACS Appl. Mater. Interfaces 2018, 10, 24310-24319; b) S. Sihler, H. Amenitsch, M. Linden, U. Ziener, Langmuir 2022, 38, 9741-9750.
News & Events
Latest Publications
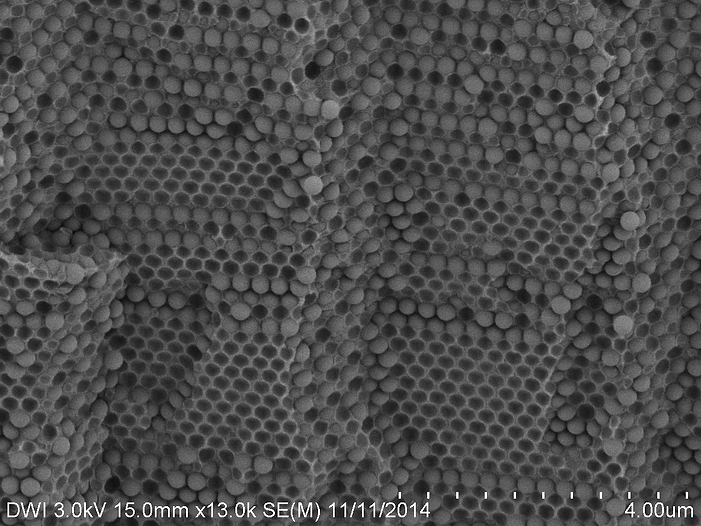